Update image
- Creighton and McClintock's experiment in corn


Support our developers
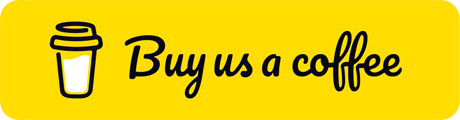
More in this section
- Linkage and Crossing Over in Diploid Organisms
- Coupling and repulsion hypothesis
- A testcross in maize
- Crossing over and meiosis
- Crossing over and chiasma formation
- Mechanism of genetic recombination
- Crossing over and linkage maps
- Recombination frequencies from a test-cross
- Recombination frequencies from F 2 data
- Interference and coincidence
- Linkage maps
- Mapping function and poisson distribution
- Linkage groups
- Chi-square test
- Cytological basis of crossing over
- Meselson and Weigle's experiment using lambda ( λ ) phage
- Crossing over at four strand stage
© 2012 - 2024 Biocyclopedia
Disclaimer Privacy Policy Feedback
Login to your account
Login / signup with.
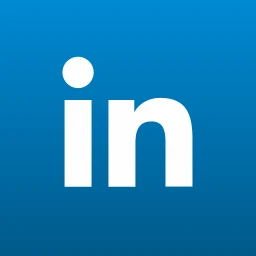
An official website of the United States government
Official websites use .gov A .gov website belongs to an official government organization in the United States.
Secure .gov websites use HTTPS A lock ( Lock Locked padlock icon ) or https:// means you've safely connected to the .gov website. Share sensitive information only on official, secure websites.
- Publications
- Account settings
- Advanced Search
- Journal List

Proof of physical exchange of genes on the chromosomes
- Author information
- Article notes
- Copyright and License information
To whom correspondence should be addressed. E-mail: [email protected] .
Communicated by Nina Fedoroff, Pennsylvania State University, University Park, PA, November 30, 2004
Received 2004 Oct 18; Issue date 2005 May 10.
Seventy-five years ago, a convincing demonstration that the genes were physically aligned along the chromosome was lacking. Harriet Creighton (1909–2004) and Barbara McClintock (1902–1992) [Creighton, H. B. & McClintock, B. (1931) Proc. Natl. Acad. Sci. USA 17, 492–497] showed by an elegantly simple experiment in 1931 that exchange between genes was accompanied by exchange of cytological, i.e., physical, parts of chromosomes. The work has been acclaimed as one of the great experiments in biology. Creighton's doctoral dissertation under McClintock's mentorship provided the basis for the landmark paper, which was unique in merging cytological with genetic data. A companion paper by McClintock, printed and bound back-to-back with the joint paper, set the essential stage with data on the cytological and genetic features that Creighton applied. Following directly from this work, and leading to today's recognition that the genome is a graspable entity, was the knowledge that the genes could be studied as components of a linear structure, the chromosome. Here, we review the data surrounding the Creighton and McClintock paper and provide a perspective on the significance of their findings.
The essential components to the demonstration of cytological and genetic crossing over are ( i ) differential features along the chromosomes that are morphologically (i.e., physically) recognizable and ( ii ) genes in the region of the cytological markers. The question is whether the order of the cytological features directly corresponds with the order of the genes as determined by their frequencies of crossing-over and recombination, that is, in a genetic map. The experiments designed by Creighton and McClintock with Zea mays ( 1 ) were paralleled by those of Stern ( 2 ) with Drosophila , published soon afterward. Creighton and McClintock introduce their report with explicit clarity as follows:
A requirement for the genetical study of crossing-over is the heterozygous condition of two allelomorphic factors [edited in ink on reprints by one of the authors to read `factor pairs'] in the same linkage group. The analysis of the behavior of homologous or partially homologous chromosomes, which are morphologically distinguishable at two points, should show evidence of cytological crossing-over. It is the aim of the present paper to show that cytological crossing-over occurs and that it is accompanied by genetical crossing-over.
Two cytological features were sufficient for the experiment: ( i ) a dark-staining, heterochromatic “knob” at the end of chromosome 9, and ( ii ) a reciprocal interchange (translocation) of a part of chromosome 9 with a part of chromosome 8 ( Fig. 1 a and b ). The knob feature is present in some strains and absent in others, while the interchange had been found as an exceptional type termed semisterile-2, studied by Burnham ( 3 ), and subsequently renamed T8-9a. The knob has no effect on the appearance of the plants, but the translocation, when heterozygous with normal chromosomes 8 and 9, results in 50% of pollen grains being sterile and empty and 50% of eggs, embryo sacs, and ovules aborting—consequently, the ear becomes only half-filled, and the kernels are irregularly distributed. This semisterility results from the formation of deficient gametes following synapsis of four chromosomes in a cross-shaped configuration ( Fig. 1 c ). This configuration opens out into a ring ( Fig. 1 d ), from which the centromeres distribute either two alternate chromosomes, with balanced (viable) genomic constitutions, or two adjacent chromosomes, with deficient-duplicate (inviable) constitutions. Thus, classification of plants for the presence of the translocation can be done either by its effects on fertility or by cytology.
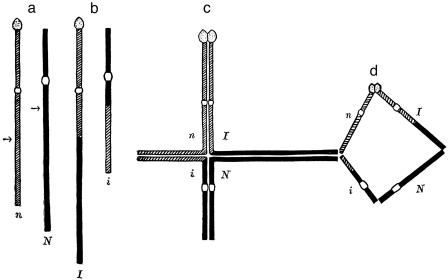
Chromosomal constitution and cytological features used in the maize experiment. Centromeres are shown as clear circles. In a , the two normal chromosomes, number 9 ( n , barred) bearing a dark-staining knob at the end of its short arm and 8 ( N , black) are shown with arrows denoting the point of reciprocal interchange of parts to form a translocation. In b , interchange chromosome 9 ( I ) has a part of chromosome 8 appended, and chromosome 8 ( i ) bears a part of chromosome 9. In c , the normal and interchanged chromosomes are shown diagrammatically in the paired cross formation in early meiosis during the process of gamete formation (see Fig. 3 ). In d , the opening out of the four chromosomes into a ring is diagrammed, from which adjacent or alternate pairs of chromosomes distribute to the division poles, resulting in deficient, inviable gametes or viable ones, respectively. The chromosomes are shown as single rather than double strands in this diagram, for simplicity. For the demonstration of crossing-over, the constitution in c and d required that one chromosome 9 end be knobbed and the other knobless. [Reproduced with permission from ref. 12 (courtesy of Peter McKinley).]
Gabriel and Fogel ( 4 ) define the context and the impact of the experiment:
The relationship between genetic recombination and the occurrence of chromatin exchange between equivalent segments of homologous chromosomes at the cytological level was first suggested by Morgan in 1911 as an explanation for the genetic phenomenon of linkage.... Morgan ingeniously emphasized that if the genes were arranged in a linear fashion on the chromosomes, equivalent blocks of maternal and paternal genes could be exchanged during the period of meiotic synapsis through the formation of chiasmata. These [chiasmata] had been described previously by Janssens [1909]. While the utility of this outlook was abundantly verified in thousands of varied experiments, and no disagreements between theory and observation were reported in the twenty-year period following its introduction, direct proof conclusively validating the theory was notably lacking. That no such proof was obtained for nearly twenty years is more easily appreciated if it is recalled that two homologous chromosomes are identical even in favorable cytological material under ideal conditions. Thus, while chiasmata are directly observable, and while they might be interpreted as due to an exchange between homologous chromosomes, there is no simple way to visually differentiate the maternal and paternal elements in an exchange.... Beyond any question, this is one of the truly great experiments of modern biology, and the conclusion—that pairing chromosomes heteromorphic for two or more regions exchange parts at the same time that they exchange genes assigned to these regions—will doubtless continue to stimulate as much research during the next as they have during the 25 years following its publication.
The experiment of Creighton and McClintock, and the parallel experiment by Stern with Drosophila published in the same year, established the commonality in linear order between the chromosome and the genes within it. Only then could it be considered likely that the genetic material extended in a linear tract from one end of the chromosome to the other. This was the point at which 20 years of concentration on the nature of the genetic material was set in motion, brought to fruition by Watson and Crick ( 5 ).
Origins of the Components of the Experiment
The morphological markers had been discovered in prior studies and were used for this experiment based on improved knowledge of their chromosome locations. This period was one of very rapid development of cytology, of genetics, and associating genes with the chromosomes that bear them, through an intricate cascade of experiments substantially conducted by McClintock at Cornell University ( 6 , 7 ). The maize chromosomes had been distinguished under the microscope by McClintock in 1929 on the basis of their relative length, arm ratios, and dark-staining knob positions. The knob at the end of chromosome 9, the second smallest chromosome, however, was first specifically described by McClintock ( 8 ) in the course of her study on “A cytological demonstration of the location of an interchange between two non-homologous chromosomes of Zea mays ” as follows:
Fortunately, the second smallest chromosome possesses, in certain strains of maize, a very conspicuous accumulation of stainable substance at the end of the short arm...
McClintock observed these chromosomes from preparations she made from a maize stock she received from Charles Burnham. The photographs she mentioned in her paper were subsequently published ( Fig. 2 a and b ) in Sharp's widely used 1934 cytology textbook ( 9 ).
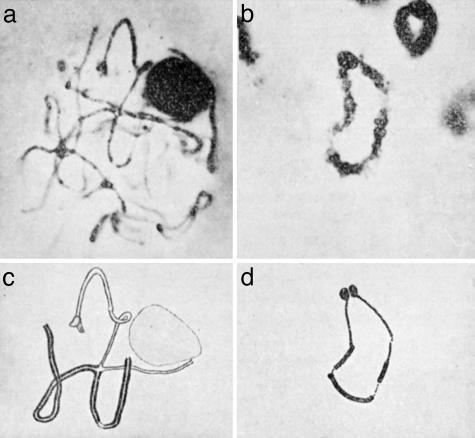
Photomicrographs of maize chromosomes carrying the 8–9 translocation, at the time of chromosome pairing ( a ) and opening out ( b ), and diagrams ( c and d ). At the stage in a , heterochromatic regions display differential staining along the lengths and between the chromosomes. a is from McClintock (not previously published), and b is from Creighton (1933). [Reproduced with permission from ref. 9 (Copyright 1934, McGraw–Hill).]
The interchange between chromosome 9 and chromosome 8 was first recognized by its effects on fertility, with 50% pollen and kernel abortion, and was named “Semisterile-2” in the studies of Brink and Burnham ( 10 , 11 ). Recognizing Belling's hypothesis of segmental interchange, they suggested that an interchange of chromosome segments could explain the genetic behavior, and Burnham ( 3 ) and McClintock ( 8 ) confirmed cytologically that two chromosomes had exchanged parts. The consequences of distribution of chromosomes after pairing of two normal chromosomes with two interchanged chromosomes, leading to 50% sterility ( Fig. 3 ), were mutually recognized by these authors.
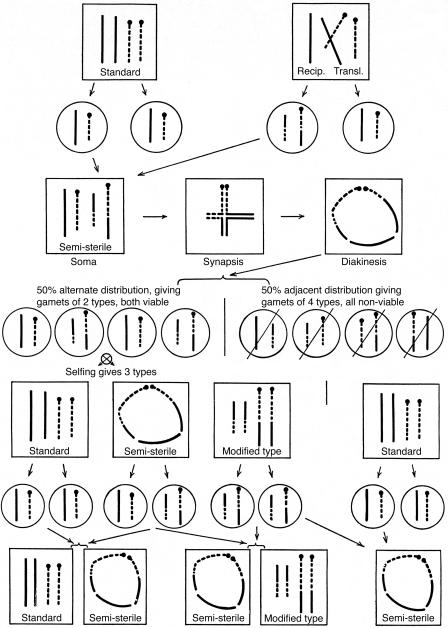
Consequences of distributions of chromosomes from a heterozygous translocation, showing the arising of viable vs. deficient-duplicate, nonviable gametes and the results of self-pollination. Note that there are three classes in such a progeny, occurring in a 1:2:1 ratio: standard chromosome constitution with normal pollen grains, heterozygous translocation with semisterile pollen grains, and homozygous translocation with normal pollen grains, due to a complete but rearranged set of chromosomes. [Reproduced with permission from ref. 9 (Copyright 1934, McGraw–Hill).]
The genetic markers of the “ C – wx ” linkage group had been mapped in relation to each other in studies by other scientists, and these data had been compiled by R. A. Emerson and G. W. Beadle in 1929 and 1930 during early cooperation among maize geneticists. Association of these linked markers with chromosome 9 was established by McClintock ( 12 ) with experiments involving an extra chromosome, and was doubly reinforced by mapping the knob and genetic markers together (see below).
The Significance of Back-to-Back Papers
The paper by Creighton and McClintock was not presented as a free-standing report. In a paper just preceding it in the journal (both were distributed as one offprint in a single cover bearing both titles), McClintock ( 12 ) set the stage with mapping data placing three genes, c, sh , and wx (respectively affecting color, shape, and starch properties of the kernel), on chromosome 9, in order with the interchange and with the knob. First, McClintock established the association of these genes with the chromosome bearing two morphological markers, the knob and the interchanged portion, in plants with an extra chromosome. The correspondence between the presence of the extra chromosome and abnormal ratios for the genes was the key determinant, and this was the point at which the association of the group of linked genes with a specific cytological chromosome, number 9, was defined. The other chromosome in the interchange, chromosome 8, was recognizable by its size and other morphological features. Semisterile-2 was thus defined as a translocation between chromosomes 8 and 9. Mapping data defined the order on chromosome 9 to be knob– c – sh – wx –interchange. The purpose of the first paper is stated in the last sentence: “ It was desired to present briefly the evidence at this time, since it lends valuable support to the argument in the paper which follows .” The paper by Creighton and McClintock refers twice to the “preceding paper” without a citation, assuming the reader will read the two together. In contrast, they refer twice to a relevant “previous paper” by McClintock ( 8 ) in the preceding year. The importance of the “preceding paper” is emphasized in an annotation in the “Current list of Barbara McClintock's publications” [L.B.K., Maize Genetics Cooperation Newsletter (1999) 73, pp. 42–48].
Creighton recalled that Thomas Hunt Morgan pushed them to publish their data (Creighton, 1982, taped symposium, courtesy of Rosalind Morris). Emerson sent both papers to PNAS and specifically asked the editor to publish them together and, if they could not, to publish the paper by McClintock first [letter from R. A. Emerson to Edwin B. Wilson, July 3, 1931, Plant Breeding Records, Rare and Manuscript Collections, Carl A. Kroch Library, Cornell University Library, Ithaca, NY (hereafter abbreviated as CU)].
Applying the information from the “preceding paper” ( 12 ) on map order of the components, Creighton and McClintock conducted an experimental cross of the constitution shown in Fig. 4 . First they presented data showing that the frequencies of recombination, ≈39% between the knob and the interchange and ≈16% between the knob and gene c , are consistent with the order and distances provided in the preceding paper. This interpretation is most important for what follows: cytological crossing over between heteromorphic chromosomes (between normal chromosome 9 and the interchange chromosome—specifically the section between the knob on the short arm of chromosome 9 and the attached piece of chromosome 8 in T8-9a) accompanying genetic crossing over between loci ( c and wx ). The table of data presented by Creighton and McClintock, reproduced here ( Fig. 5 ), was accompanied by this comment: “ The data are necessarily few since the ear contained but few kernels .” Consideration of each individual plant of the progeny is followed promptly in the paper by a one-sentence final statement and conclusions:
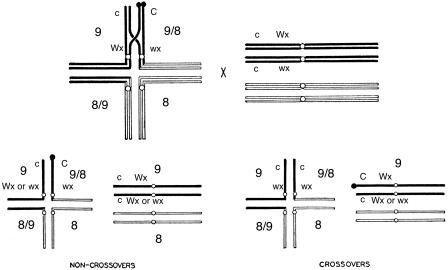
The experimental maize cross and progeny types designed to test the hypothesis that exchange between genes is accompanied by exchange of physical parts of chromosomes. ( Upper ) a morphologically marked hybrid, knobbed vs. knobless and normal vs. interchanged, and a genetically marked hybrid, C (colored kernels) vs. c (colorless kernels) and Wx (normal starch, blue-staining kernels and pollen) vs. wx (waxy starch, reddish-staining seeds and pollen) is crossed with knobless normal chromosomes bearing c and heterozygous for Wx and wx . One crossover in the critical region is shown in the hybrid, exchanging parts between the genes and between the physical markers. ( Lower ) the (viable) products resulting from no crossover or a crossover in the critical region. Note that, because the pollen can be classified for Wx vs. wx , progeny plants that are Wx Wx vs. Wx wx vs. wx wx can be distinguished by staining samples of the pollen. Note also that, because plants heterozygous for a translocation have 50% aborted pollen and eggs (Fig. 4), progeny plants can be classified for normal vs. interchange-carrying constitution. (Adapted from ref. 13 to reflect the exact experiment.)
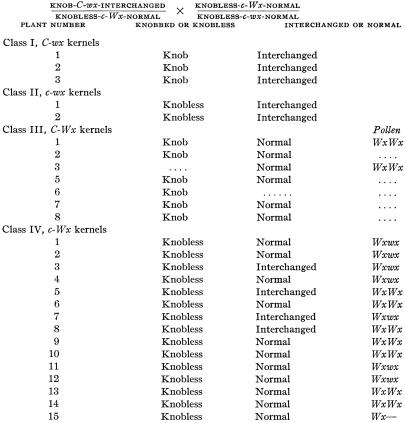
Data presented by Creighton and McClintock, 1931, Table 3. Kernels were classified as C wx (colored waxy kernels, non-crossover—see Fig. 4 ), cwx (colorless waxy kernels, crossover), CWx (colored non-waxy kernels, crossover), and cWx (colorless non-waxy kernels, non-crossover). Progeny plants may be classified for the knob by cytology at meiosis or in postmeiotic divisions, for the interchange by cytology or by semisterile vs. normal pollen, and for wx constitution by staining samples of pollen with an iodine solution (IKI), as described in Fig. 4 . Plants from Class I carried the knob and the interchange, that is, without genetic or physical exchange, while those from Class II were knobless and carried the interchange, that is, with a physical exchange accompanying the genetic exchange. In Class III, those individuals that could be classified showed a physical crossover and accompanying genetic exchange. In Class IV, all of the individuals were knobless (having no exchange between the knob and gene c ), and those without physical exchange (Knobless and Normal) were consistent with no genetic exchange, while those with physical exchange (Knobless and Interchanged) were due either to genetic exchanges in the critical region or between wx and the interchange. [Reproduced with permission from ref. 1 (courtesy of Peter McKinley and the Creighton estate).]
The foregoing evidence points to the fact that cytological crossing-over occurs and is accompanied by the expected types of genetic crossing-over. Conclusions.—Pairing chromosomes, heteromorphic in two regions, have been shown to exchange parts at the same time they exchange genes assigned to these regions.
Notably, the “preceding paper” by McClintock relates and cites data from two then-current sources of shared data: A “Mimeographed pamphlet on linkage in maize. Cornell University” (published and unpublished data that were compiled and distributed to colleagues by R. A. Emerson as a part of the nascent Maize Genetic Cooperation in 1929 and 1930, reprinted in Maize Genetics Cooperation Newsletter 53 and 54, respectively—L.B.K. and E.C., unpublished work) and “Unpublished data which Dr. C. R. Burnham has generously allowed me to use...” The latter data were subsequently published by Burnham in 1934 ( 14 ), accompanied by other data furnished by Creighton, and the data of both were partly incorporated in the monographic 1935 “A Summary of Linkage Studies in Maize” by Emerson, Beadle, and Fraser ( 15 ). In this vein, we offer the following informative correspondence relevant to shared data and cooperation among scientists.
Parts of the Data Were Unpublished Work Shared by Charles R. Burnham
McClintock asked Burnham to coauthor the paper on the order of the genes (McClintock to Burnham, May 12, 1931, in the papers of C. R. Burnham, University of Minnesota archives):
P.S. Would you care to write up the order of the genes of the c-sh-wx linkage group with me? I think it ought to be done soon and should go in the Proc-Nat Acad-Sci. Your unpublished data is [sic] as essential as mine. Let me know what you think.
McClintock told Provine ( 16 ) that she first had to show the order of the genes before she could publish the crossing-over data. On June 11, 1931, McClintock wrote to Burnham and enclosed her manuscript on the order of the genes:
Sharp, Rhoades, & Stadler have worked it over.... The paper which goes with it is Harriet's—`A correlation of cytological & genetic crossingover.' I am growing more material to make the evidence wider in scope but I believe that this is not necessary for this paper. The part marked across the page is the part which you are to do as you like with. I don't know whether you want to put in a table, make a statement or what. The figures I obtained from a letter you wrote in December. [The letter has not been located.] Maybe there is more data now. I think you will see what ought to be put in here to give the meaning intended. If you do not want to go in with me I should feel bad for your data has been a key for me to work with. You may not want to take the responsibility since you were not in close touch with this latter work when it was done. I hope you won't feel that way although be frank about it & say what you please. Don't injure yourself to please me. I am anxious to get Harriet's data in press as I have been advised to push it. [See story about Morgan pushing them to publish in Kass ( 17 ), per Creighton (1982), taped symposium, courtesy of Rosalind Morris. Morgan gave the Messinger Lectures at Cornell in March/April of 1931, before publication of the subject paper (CU) ( 18 ).] Since this paper goes first [emphasis added] in the same issue of the Proceedings of the National Academy of Science I am anxious to get it off as soon as possible. I don't know how busy you are now & whether you can spare a few hours during the next few days to do it. I hope so. Let me know by a short note what the situation is so that I will know what to expect.
Two weeks later McClintock wrote to Burnham (June 26, 1931) expressing her disappointment with his decision not to publish collaboratively:
I was much disappointed that you feel you can't go in with me on the paper. The reasons you gave I do not think are strong enough. I would be glad if you change your mind. I don't want to force you, so you do as you please. I will go ahead now but can insert any changes or additions later if you will come in with me.... I saw Robbins of the National Research Council. He said the joint authorship need not hold up the paper so that reason is dispensed with. You can let me know any time soon.
The appreciation is encompassed in their crossing-over paper with a simple acknowledgment that follows their final statement and the conclusions:
[The authors] are indebted to Dr. C. R. Burnham for furnishing unpublished data and for some of the material studied.
Creighton's Role
Harriet Creighton carried out this widely cited crossing-over experiment as part of her 1933 doctoral thesis ( 19 ), in which she acknowledged “ indebtedness to Dr. Barbara McClintock for her aid and constant encouragement during the course of this study, which she suggested .” Therein, she summarized the background papers by McClintock ( 8 ) and Burnham ( 3 ) and included photographs that had been taken by Barbara McClintock. She specifically used the microscopic photograph of maize showing eight paired chromosomes and a ring of four chromosomes, carrying the 8–9 translocation and opening out at diakinesis (late prophase of meiosis). A close-up of the latter ( Fig. 2 b and d ) was published by her major professor L. W. Sharp ( 9 ) along with the photograph by McClintock mentioned above ( Fig. 2 a and c ; see also Fig. 1 d ). It was this stage of the division process that played an important part in their cytological observations in 1931: “ It is clear that these two chromosomes which synapse along their homologous parts during prophase of meiosis in the resulting individual are visibly different at each of their two ends ” ( 1 ). Creighton (interview by L.B.K., August 23, 1994) recalled in 1994 that because of her inexperience she really did not appreciate the significance of the study when it was initially proposed to her by McClintock. She was also quite surprised when she learned recently that their paper had been so widely cited. [A cited reference search for their 1931 publication in the Science Citation Index Expanded (1945 to the present) ( 20 ) recovered 61 citations between 1945 and 2004. By way of contrast, the companion paper by McClintock ( 12 ) elicited 12 citations. Stern's 1931 crossing-over paper ( 2 ), which appeared within months of Creighton and McClintock's paper, was cited 38 times during this same time span—27 citations overlapping with those of Creighton and McClintock.]
Concise Reports of High Quality
Perhaps because the most essential background information was presented in a separate but companion paper, and likely because of confusion between the words “preceding paper” and “previous paper,” some subsequent writers, while crediting it with its place in biological history, have considered the report difficult to absorb. For example, Robbins ( 21 ), commenting with the reprinting in the Classical Genetics papers of Electronic Scholarly Publishing ( www.esp.org ), states that the authors “do not take much time to help readers understand the underlying logic or to appreciate the subtleties of their analysis.” Peters ( 22 ), reprinting the paper among a selection of classics, comments, “This paper has been called a landmark in experimental genetics. It is more than that—it is a cornerstone. It is not an easy paper to follow, for the items that require retention throughout the analysis are many, and it is fatal to one's understanding to lose track of any of them.” The experiment is very widely covered in textbooks and histories, although admittedly most presentations are over-simplified or inaccurate. The focus of the crossing-over paper was placed on the specific theoretical issue by defining the elements of the experiment in the “preceding” paper by McClintock ( 12 ). The experiment, in fact, was exemplary and elegant in its simplicity.
With regard to the brevity of the paper, the fact is that the PNAS was a forum to get new results published quickly and was not supposed to include all details of the investigations. Articles were originally limited to six (small) pages and occasionally went over this limit when funds were available. This is clear from the correspondence in Emerson's files at Cornell. On December 14, 1933, Edwin B. Wilson wrote to Emerson (CU) about a subsequent article he had sent for Creighton:
I am afraid it will set more than 6 pages.... I am wondering how we can modify this so that it will set under 6 pages.... [Around 1925–26] a special grant became available to enlarge the size of the Proceedings and take some articles in excess of 6 pages provided they were still short. The money... has been spent.... The reason we have to go back to 6 pages is that the old rule of the Proceedings.... limited articles to those which the Managing Editor thought would set within 6 pages. The idea was in a mixed journal it was important to have a variety of sciences represented.
Wilson goes on to suggest limiting papers to members of the academy if longer than six pages. He explains why he has to reject articles over six pages, and ends with an appeal to discuss the matter with other members of the Academy at Cornell. Emerson replied on December 18 that he was returning Creighton's revised manuscript
... within the six page limit.
He added that he would like to get
prompt publication of brief papers of some of my students and former students who are, as it happens, turning out relatively important contributions in the field of cyto-genetics.
Wilson wrote to Emerson on December 20, 1933 (CU), from the Office of Editors of the PNAS, regarding limiting the Proceedings to papers that
... come under the very carefully written rule which is in the second paragraph of our information to contributors on the 3 rd page of our cover... It wanted little philosophic specific articles showing the relation of the new discoveries to previous work of the authors and others and if possible their implication for other branches of science or for future work. They wanted some evidence of how the work was obtained but specifically said that elaborate tables and graphs and the description of details should not be permitted.... there was every desire to avoid competition with purely professional technical journals.... I note you want the proceedings kept open to non-members and I interpret this to mean that of the two alternatives for cutting... that we should go back strictly to our 6 page limit but not ex-clude non-members.... In other words, the university people will want to include the best work of their best students...
The parallel experiment by Stern with Drosophila , also published in 1931, involved a translocation on one side of a gene-marked segment, and a differential broken chromosome on the other. Sharp ( 9 ), in displaying the two experiments side-by-side ( Fig. 6 ), demonstrates the cunning simplicity of each experiment. The pared-down diagram presented by Creighton and McClintock ( Fig. 7 ) displayed only the essential components of the cross, excluding nonessential complementary segments.
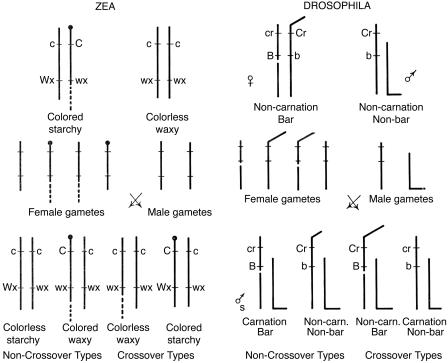
Comparative diagrams of the experiments of Creighton and McClintock with maize and of Stern with Drosophila , mutually supporting the hypothesis of physical and genetic exchange in two key experimental species. [Reproduced with permission from ref. 9 (Copyright 1934, McGraw–Hill).]

Simplified diagram of the cross as presented by Creighton and McClintock, 1931, as a text diagram (p. 495). Note that in this diagram, interchanged chromosome 9 ( I ) carries the knob and normal chromosome 9 ( N ) is knobless. Centromeres are not identified, and nonessential segments are excluded. [Reproduced with permission from ref. 1 (courtesy of Peter McKinley and the Creighton estate).]
It may seem surprising that 20 years elapsed between the suggestion by Morgan that chromosomes might exchange physical parts as part of the exchange of genes, and the tests of his suggestion. Morgan was unquestionably an influential leader in genetic thinking and experimentation; so one might ask, “What deferred the appropriate experiment?” The answer lies in biological components, which had to be discovered or defined first. The technology for microscopic study of chromosomes was already well developed but was in need of refinements for viewing individual haploid and paired maize chromosomes, for example by McClintock ( 8 , 23 ). As specific cytological markers, knobs were first described in the study of McClintock ( 8 ); reciprocal translocations were recognized in Drosophila by Painter and Muller ( 24 ) and by Sturtevant and Dobzhansky ( 25 ), and in maize by Burnham ( 3 ) and McClintock ( 8 ). Extensive gene-marked segments were available in Drosophila by 1916 ( 26 ), and in maize by 1929 ( 15 ). What was required, as in most groundbreaking experiments, was the intersection of tools with knowledge, and their ingenious combination. The experiments both in Drosophila and in maize, the two principal genetic species of the time, attained the intersection at nearly the same time and were mutually reinforcing. The remarkable nature of reinforcement of proof in experimental science is that it sometimes advances in coincidences and sometimes in competition, rarely by sheer volume of data.
Acknowledgments
E.C. is grateful for the appreciation of history and of open sharing of data among all in the Maize Genetics Cooperation community, and for those who have been mentors to him in the past, especially C. R. Burnham, J. R. Laughnan, M. M. Rhoades, E. G. Anderson, G. W. Beadle, M. T. Jenkins, and G. F. Sprague. L.B.K. acknowledges the National Science Foundation (Grants SBR9511866 and SBR9710488) for support of archival research; the Departments of Plant Biology and Plant Breeding at Cornell University for logistical support, with grateful thanks to Royse P. Murphy and Harriet B. Creighton for helpful discussions and leads to documents; W. B. Provine for use of his reprint collection; E. B. Lewis for use of the Morgan reprint collection at the California Institute of Technology; Rosalind Morris for sharing her tape of the 75th Synapsis Club Reunion at Cornell University; Librarians at Mann Library, Olin Library, and the L. H. Bailey Hortorium Library, Cornell University; and archivist at the University of Minnesota Archives and the Division of Rare and Manuscript Collections, Carl A. Kroch Library, Cornell University, for permission to use and cite from the collections. Special thanks to our colleagues Michael McMullen and Charles Uhl for helpful suggestions on revising the manuscript.
- 1. Creighton, H. B. & McClintock, B. (1931) Proc. Natl. Acad. Sci. USA 17 , 492–497. [ DOI ] [ PMC free article ] [ PubMed ] [ Google Scholar ]
- 2. Stern, C. (1931) Biol. Zent. Bl. 51 , 547–587. [ Google Scholar ]
- 3. Burnham, C. R. (1930) Proc. Natl. Acad. Sci. USA 16 , 269–277. [ DOI ] [ PMC free article ] [ PubMed ] [ Google Scholar ]
- 4. Gabriel, M. & Fogel, S. (1955) Great Experiments in Biology (Prentice–Hall, Englewood Cliffs, NJ), pp. 267–268.
- 5. Watson, J. D. & Crick, F. H. C. (1953) Nature 171 , 737–738. [ DOI ] [ PubMed ] [ Google Scholar ]
- 6. Rhoades, M. M. & McClintock, B. (1935) Bot. Rev. 1 , 292–325. [ Google Scholar ]
- 7. Kass, L. B. & Bonneuil, C. (2004) in Classic Genetic Research and Its Legacy: The Mapping Cultures of 20th Century Genetics, eds. Rheinberger, H.-J. & Gaudilliere, J.-P. (Routledge, London), pp. 91–118.
- 8. McClintock, B. (1930) Proc. Natl. Acad. Sci. USA 16 , 791–796. [ DOI ] [ PMC free article ] [ PubMed ] [ Google Scholar ]
- 9. Sharp, L. (1934) Introduction to Cytology (McGraw–Hill, New York), pp. 303, 330, 333.
- 10. Brink, R. A. (1927) J. Hered. 18 , 266–270. [ Google Scholar ]
- 11. Brink, R. A. & Burnham, C. R. (1929) Am. Nat. 63 , 301–316. [ Google Scholar ]
- 12. McClintock, B. (1931) Proc. Natl. Acad. Sci. USA 17 , 485–491. [ DOI ] [ PMC free article ] [ PubMed ] [ Google Scholar ]
- 13. Sturtevant, A. & Beadle, G. (1939) An Introduction to Genetics (Saunders, Philadelphia), pp. 185–186.
- 14. Burnham, C. R. (1934) Genetics 19 , 430–447. [ DOI ] [ PMC free article ] [ PubMed ] [ Google Scholar ]
- 15. Emerson, R. A., Beadle, G. W. & Fraser, A. C. (1935) Cornell Univ. Agric. Exp. Stn. Mem. 180 , 1–83. [ Google Scholar ]
- 16. Provine, W. B. & Sisco, P. (1980) Interview with Barbara McClintock at Cold Spring Harbor Laboratory, Long Island, New York (Division of Rare and Manuscript Collections, Carl A. Kroch Library, Cornell Univ. Library, Ithaca, NY).
- 17. Kass, L. B. (2003) Genetics 164 , 1251–1260. [ DOI ] [ PMC free article ] [ PubMed ] [ Google Scholar ]
- 18. Morgan, T. H. (1932) The Scientific Basis of Evolution (Norton, New York).
- 19. Creighton, H. B. (1933) Ph.D. thesis (Cornell Univ., Ithaca, NY).
- 20. Science Citation Index ISI Web of Science/Science Citation Index Expanded (2004) http://isi4.isiknowledge.com/portal.cgi . Accessed September 24, 2004.
- 21. Robbins, R. J. (2003) www.esp.org/foundations/genetics/classical/holdings/m/hc-bm-31.pdf . Accessed September 15, 2004.
- 22. Peters, J. A. (1959) Classic Papers in Genetics (Prentice–Hall, Englewood Cliffs, NJ), pp. 155–156.
- 23. McClintock, B. (1929) Science 69 , 629. [ DOI ] [ PubMed ] [ Google Scholar ]
- 24. Painter, T. S. & Muller, H. J. (1929) J. Hered. 20 , 287–298. [ Google Scholar ]
- 25. Sturtevant, A. & Dobzhansky, T. (1930) Proc. Natl. Acad. Sci. USA 16 , 533–536. [ DOI ] [ PMC free article ] [ PubMed ] [ Google Scholar ]
- 26. Bridges, C. B. (1916) Genetics 1 , 1–52. [ DOI ] [ PMC free article ] [ PubMed ] [ Google Scholar ]
- View on publisher site
- PDF (494.3 KB)
- Collections
Similar articles
Cited by other articles, links to ncbi databases.
- Download .nbib .nbib
- Format: AMA APA MLA NLM
Add to Collections
Barbara McClintock's Transposon Experiments in Maize (1931–1951)
Barbara McClintock conducted experiments on corn ( Zea mays ) in the United States in the mid-twentieth century to study the structure and function of the chromosomes in the cells. McClintock researched how genes combined in corn and proposed mechanisms for how those interactions are regulated. McClintock received the Nobel Prize in Physiology or Medicine in 1983, the first woman to win the prize without sharing it. McClintock won the award for her introduction of the concept of transposons, also called jumping genes. McClintock conceptualized some genetic material as not static in structure and order, but as subject to re-arrangement and may be altered during development.
McClintock began her research into transposons by examining how genes combined in a process called crossing over. Prior to McClintock's research, scientists had studied crossing-over. Crossing over is the process by which homologous chromosomes align side by side during meiosis and physically exchange genetic material. In 1909 Frans Alfons Janssens in Belgium described the phenomenon of chromosomal re-arrangement as chiasmatypie , a process in which chromosomes arrange in the shape of an X, the center of which became called the chiasma after chi in the Greek alphabet. Then in 1916 Thomas Hunt Morgan working on genetics in his fly lab at Columbia University in New York City, New York, hypothesized that chromosomes go through a crossing-over process during development. In 1931, McClintock built on that research using corn plants to provide a description of the physical basis of chromosomal crossing-over.
McClintock began her work on genetic recombination and transposition with doctoral student Harriet Baldwin Creighton in 1931 at Cornell's College of Agriculture in Ithaca, New York. Together they examined maize chromosomes and published their results in the 1931 paper "A Correlation of Cytological and Genetical Crossing-Over in Zea Mays ." In that paper, they described the physical basis for the exchange of genetic material between homologous chromosomes, called crossing-over. McClintock continued her research solo, and in 1944 at Cold Spring Harbor Laboratory in Laurel Hollow, New York, she began her experiments to test the existence of some genetic elements on the chromosome that are capable of movement. She labeled those elements as transposable elements or transposons, and she published on the findings in 1950's "The Origin and Behavior of Mutable Loci in Maize". The discovery of transposons provided a causal explanation for unusual phenotypic features in maize after breeding and helped to identify the mobile elements on chromosomes.
McClintock's study of transposable elements on chromosomes attempted to clarify Rollins Adams Emerson's 1910 research about the occurrence of purple or brown spots on white kernels in maize, called colorless. Emerson had hypothesized that the spots occurred due to unstable mutations on chromosomes. Yet, Emerson had not provide evidence for that explanation of the phenomenon. McClintock instead suggested that the occurrence of unexpected purple- and brown-colored kernels was due to genetic transposition.
To test that claim, McClintock bred maize plants to vary in the color of the kernels. McClintock took cell samples from the corn, dyed the samples, looked at them under the microscope by using staining techniques to enhance the contrast in the microscopic images, and observed the chromosomes. McClintock bred maize plants carrying chromosomes that resulted in a recessive brown phenotype (called bz) with maize plants with chromosomes that coded for a dominant white phenotype (called colorless or C'). Given normal theories of inheritance, the offspring should have been maize plants displaying white kernels, but instead those kernels were white with some brown spots. McClintock attributed the unexpected variation to a chromosomal breakage in which a chromosome lost one allele. McClintock called that phenomenon dissociation, which occurred at a chromosomal locus she called Ds . She hypothesized that the kernels carrying three alleles must have lost both their dominant colorless allele and their dominant purple allele due to breakage, and the loses resulted in the occurrence of some recessive brown kernels among the expected colorless kernels. McClintock called the chromosomal site where that breakage supposedly occurred, dissociation ( Ds ). Additionally, she argued that such a breakage took place due to a factor called activator (Ac). The amount of brown spots on the creamy background depended on the timing of breakage during development.
When McClintock presented her findings at a Cold Spring Harbor Symposium in 1951, the audience at the conference considered her results to be obscure and difficult to understand. McClintock's research did not fit with then current theories of genetic phenomena, as genetic material was conceived as a static entity before the 1960s. Thus, scientists paid little attention to McClintock's experiments before François Jacob and Jacques Monod described similar phenomena in bacteria in 1960.
Decades later in 1983, McClintock received the Nobel Prize in Physiology or Medicine for her experiments detailing the evidence for transposons. McClintock's work did not receive widespread recognition among scientists until the 1980s, which provoked a debate. According to Evelyn Fox Keller, an historian of biology, McClintock was awarded the Nobel Prize more than thirty years after the publication of her results due to gender inequalities in science. Contrary to Fox Keller's interpretation, historian of science Nathaniel Comfort argued that McClintock had to wait so long before receiving scientific acclaim because some aspects of genetics were still unclear until the early 1980s, and therefore McClintock's research required a more comprehensive framework to validate her results.
In the 1950s, McClintock could not test her hypothesis about controlling elements, like Ds and Ac, because the structure of the DNA molecule had not yet been discovered at the time of her research, so it was difficult to locate those elements. Additionally, the genetic techniques at the time when McClintock conducted her research could not identify or intervene in specific parts of the genome. The existence of transposable elements was finally tested in the early 1980s, when Nina Fedoroff and her team isolated and cloned the elements.
McClintock's experiments on maize plants highlighted the instability of genetic material and stated the existence of transposable elements in the genome.
- Comfort, Nathaniel C. "From Controlling Elements to Transposons: Barbara McClintock and the Nobel Prize." Trends in Genetics 17 (2001): 475–8. http://www.sciencedirect.com/science/article/pii/S0968000401018989 (Accessed December 8, 2015).
- Craig, Patricia P. Jumping Genes: Barbara McClintock's Scientific Legacy: An Essay about Basic Research from the Carnegie Institution of Washington . Washington D.C.: Carnegie Institution, 1994.
- Emerson, Rollins Adams. "Latent colors in corn." Journal of Heredity 1 (1911): 233–237.
- Fedoroff, Nina, Susan Wessler, and Mavis Shure. "Isolation of the Transposable Maize Controlling Elements Ac and Ds." Cell 35 (1983): 235–42. http://www.cell.com/cell/abstract/0092-8674(83)90226-X?_returnURL=http%3A%2F%2Flinkinghub.elsevier.com%2Fretrieve%2Fpii%2F009286748390226X%3Fshowall%3Dtrue (December 8, 2015).
- Federoff, Nina, and David Botstein. The Dynamic Genome: Barbara McClintock's Ideas in the Century of Genetics . Cold Spring Harbor: Cold Spring Harbor Laboratory Press, 1992.
- Fox Keller, Evelyn. A Feeling for the Organism . New York: W. H. Freeman and Company, 1983.
- Janssens, François Alphonse. "La théorie de la chiasmatypie: Nouvelle interpretation des cinéses de maturation".[The chiasmatype theory. A new interpretation of the maturation divisions]. Cellule [Cell]. 25 (1909): 389–411.
- McClintock, Barbara, and Harriett B. Creighton. "A Correlation of Cytological and Genetical Crossing-Over in Zea Mays." Proceedings of the National Academy of Sciences 17 (1931): 492–7. http://www.pnas.org/content/17/8/492.full.pdf (Accessed December 8, 2015).
- McClintock, Barbara. "The Origin and Behavior of Mutable Loci in Maize." Proceedings of the National Academy of Sciences 36 (1950): 344–55. http://www.pnas.org/content/36/6/344.full.pdf (Accessed December 8, 2015).
- McClintock, Barbara. "Chromosome organization and genic expression." Cold Spring Harbor symposia on quantitative biology 16 (1951): 13–47.
- Morgan, Thomas Hunt. A Critique of the Theory of Evolution . Princeton: Princeton University Press, 1916.
- Pardee, Arthur B., François Jacob, and Jacques Monod. "The Genetic Control and Cytoplasmic Expression of "Inducibility" in the Synthesis of β-galactosidase by E. coli." Journal of Molecular Biology 1.2 (1959): 165–78. http://www.sciencedirect.com/science/article/pii/S0022283659800450 (Accessed December 8, 2015).
- Pray, Leslie, and Kira Zhaurova. "Barbara McClintock and the Discovery of Jumping Genes (Transposons)." Nature Education 1 (2008): 169. http://www.nature.com/scitable/topicpage/barbara-mcclintock-and-the-discovery-of-jumping-34083 (Accessed December 8, 2015).
- Ravindran, Sandeep. "Barbara McClintock and the Discovery of Jumping Genes." Proceedings of the National Academy of Sciences 109 (2012): 20198–99. http://www.pnas.org/content/109/50/20198.full.pdf (Accessed December 8, 2015).
How to cite
Articles rights and graphics.
Copyright Arizona Board of Regents Licensed as Creative Commons Attribution-NonCommercial-Share Alike 3.0 Unported (CC BY-NC-SA 3.0)

Last modified
Share this page.
This page has been archived and is no longer updated
Barbara McClintock and the Discovery of Jumping Genes (Transposons)

McClintock and the Origins of Cytogenetics
Barbara McClintock began her scientific career at Cornell University, where she pioneered the study of cytogenetics-a new field in the 1930s-using maize as a model organism . Indeed, the marriage of cytology and genetics became official in 1931, when McClintock and graduate student Harriet Creighton provided the first experimental proof that genes were physically positioned on chromosomes by describing the crossing-over phenomenon and genetic recombination . Although Thomas Hunt Morgan was the first person to suggest the link between genetic traits and the exchange of genetic material by chromosomes, 20 years elapsed before his ideas were scientifically proven, largely due to limitations in cytological and experimental techniques (Coe & Kass, 2005). McClintock's own innovative cytogenetic techniques allowed her to confirm Morgan's ideas, and these techniques are numbered among her greatest contributions to science.
Discovering TEs Through Experimentation with Maize
To better grasp this idea, consider every maize kernel as a single individual, originating as an ovule that has undergone double fertilization (Figure 1). During double fertilization, one sperm fuses with the egg cell 's nucleus , producing a diploid zygote that will develop into the next generation. Meanwhile, the other sperm fuses with the two polar nuclei to form a triploid endosperm, which forms an outer protein layer known as the aleurone layer. As a result, the colored (or colorless, as the case may be) tissue that makes up the aleurone layer of the kernel is triploid, not diploid.
The Ac/Ds System of Transposable Elements

McClintock worked with what is known as the Ac / Ds system in maize, which she discovered by conducting standard genetic breeding experiments with an unusual phenotype . Through these experiments, McClintock recognized that breakage occurred at specific sites on maize chromosomes. Indeed, the first transposable element she discovered was a site of chromosome breakage, aptly named "dissociation" ( Ds ). Although McClintock eventually found that some TEs can "jump" autonomously, she initially noted that the movements of Ds are regulated by an autonomous element called " activator " ( Ac ), which can also promote its own transposition .
Of course, these discoveries were preceded by extensive breeding experimentation. It was known at the time from previous work by Rollins A. Emerson, another American maize geneticist and the "rediscoverer" of Mendel's laws of inheritance , that maize had genes encoding variegated, or multicolored, kernels; these kernels were described as colorless (although they were actually white or yellow), except for spots or streaks of purple or brown (Figure 2). Emerson had proposed that the variegated streaking was due to an "unstable mutation ," or a mutation for the colorless phenotype that would sometimes revert back to its wild-type variant and result in an area of color. However, he couldn't explain why or how this occurred. As McClintock discovered, the unstable mutation Emerson puzzled over was actually a four-gene system, as outlined in Table 1.
Table 1: Maize Genes Studied by Barbara McClintock
Adapted from McClean, 1997
In her experiments, McClintock bred females that were homozygous for C and bz and that lacked Ds (denoted CCbzbz --, where the dashes indicate the absence of Ds alleles) with males that were homozygous for C' , Bz , and Ds (denoted C'C'BzBzDsDs ) to yield heterozygotes with an aleurone layer that had the genotype C'CCBzbzbz -- Ds. (Remember, in double fertilization, the sperm provides one set of alleles, and the egg provides two.) Because of the presence of the dominant inhibitor allele C' , the offspring kernels were expected to be colorless, no matter what their genetic makeup at the Bz/bz locus . In fact, upon crossbreeding, many of these kernels were indeed colorless. However, McClintock also observed many kernels with colorless backgrounds and varying amounts of dark brown spots or streaks, and she concluded that individual cells in those kernels had lost their C' and Bz alleles because of a chromosomal break at the Ds locus. Without either the C' allele (to prevent color expression) or the Bz (purple) allele, the cells that had experienced a breakage at the Ds locus ended up with some brown coloring.
Within the affected seeds, the amount of colored streaking or spotting depended upon when during seed development the somatic cell mutation at Ds occurred. If this mutation occurred early in development, then, as the one mutant cell continued to divide, more cells in the mature kernel would have the brownish phenotype, and the spot or streak of color on the kernel would be larger. On the other hand, if the mutation occurred later in development , the spotting would be smaller, because the kernel would undergo less cell division prior to maturity.
Expression of Ds in Maize
McClintock also performed additional experiments to demonstrate that the phenotypic effect of Ds depended upon the presence of another element, which she called Ac . McClintock had trouble mapping both the Ac and Ds elements, however, noting that they changed their positions on the chromosome in different maize plants. In fact, further experiments showed that Ds didn't just break chromosomes, but it could actually move from one chromosomal location to another. When Ds inserts itself into the Bz allele, for example, it causes a mutation in the Bz gene (but only when Ac is present), thereby destroying the ability of the Bz gene to produce any pigment at all. Ds can also excise from the Bz allele (again, only in the presence of Ac ), causing Bz to revert back to its purple or brown phenotype. Again, the amount of purple or brown depends upon when during development Ds is inserted or excised. If excision happens prior to fertilization, then the affected kernel will be entirely purple or brown, depending upon the Bz/bz genotype.
Years after McClintock discovered the Ac/Ds system, scientists were finally able to study both TEs in much more molecular detail. Today, we know that Ac elements are about 4,500 base pairs long and are similar in structure to other DNA transposons .
McClintock and the Theory of Epigenetics
References and recommended reading.
- Add Content to Group
Article History
Flag inappropriate.


Email your Friend

- | Lead Editor: Bob Moss

Within this Subject (34)
- Applications in Biotechnology (4)
- Discovery of Genetic Material (4)
- DNA Replication (6)
- Gene Copies (5)
- Jumping Genes (4)
- RNA (7)
- Transcription & Translation (4)
Other Topic Rooms
- Gene Inheritance and Transmission
- Gene Expression and Regulation
- Nucleic Acid Structure and Function
- Chromosomes and Cytogenetics
- Evolutionary Genetics
- Population and Quantitative Genetics
- Genes and Disease
- Genetics and Society
- Cell Origins and Metabolism
- Proteins and Gene Expression
- Subcellular Compartments
- Cell Communication
- Cell Cycle and Cell Division

© 2014 Nature Education
- Press Room |
- Terms of Use |
- Privacy Notice |

Visual Browse
Proof of physical exchange of genes on the chromosomes
Affiliation.
- 1 Agricultural Research Service-U.S. Department of Agriculture, Plant Genetics Research Unit, and Plant Sciences Unit, University of Missouri, Columbia, MO 65211, USA. [email protected]
- PMID: 15867161
- PMCID: PMC1100733
- DOI: 10.1073/pnas.0407340102
Seventy-five years ago, a convincing demonstration that the genes were physically aligned along the chromosome was lacking. Harriet Creighton (1909-2004) and Barbara McClintock (1902-1992) [Creighton, H. B. & McClintock, B. (1931) Proc. Natl. Acad. Sci. USA 17, 492-497] showed by an elegantly simple experiment in 1931 that exchange between genes was accompanied by exchange of cytological, i.e., physical, parts of chromosomes. The work has been acclaimed as one of the great experiments in biology. Creighton's doctoral dissertation under McClintock's mentorship provided the basis for the landmark paper, which was unique in merging cytological with genetic data. A companion paper by McClintock, printed and bound back-to-back with the joint paper, set the essential stage with data on the cytological and genetic features that Creighton applied. Following directly from this work, and leading to today's recognition that the genome is a graspable entity, was the knowledge that the genes could be studied as components of a linear structure, the chromosome. Here, we review the data surrounding the Creighton and McClintock paper and provide a perspective on the significance of their findings.
Publication types
- Historical Article
- Research Support, Non-U.S. Gov't
- Research Support, U.S. Gov't, Non-P.H.S.
- Chromosomes / ultrastructure*
- Crosses, Genetic
- Genes, Plant
- Genetics / history
- Heterozygote
- History, 20th Century
- Models, Genetic
- Periodicals as Topic
- Translocation, Genetic*
- United States
- Zea mays / genetics
From Stable Chromosomes to Jumping Genes: The work of Barbara McClintock
by Anne E. Egger, Ph.D., Jessica Ruvinsky, Ph.D.
Did you know that some DNA “jumps” from cell to cell, and this small percentage may be linked to genetic disorders in humans such as hemophilia, leukemia and breast cancer? “Jumping genes” were the discovery of Barbara McClintock, a Nobel-prize winning biologist whose work challenged the long-held notion of a stable genome.
Science is based on data , and collecting lots of data often involves a lot of diligent work and attention to detail. In the study of genetics and heredity , for example, scientists from Gregor Mendel in the 1800s to Craig Venter in the 2000s have spent countless hours collecting data: carefully breeding pea plants and describing their offspring or combing through millions of DNA base pairs to sequence the human genome . But even the most carefully and diligently collected data does not interpret itself. Scientists must also interpret that data, and to do so, they need to be able to see both the details and the big picture, and be creative in their thinking. Barbara McClintock (Figure 1), a Nobel Prize-winning biologist, was renowned for her sometimes obsessive attention to detail in her observations and experiments , but her work is remembered today because of the creative leaps she was able to take to explain those details.
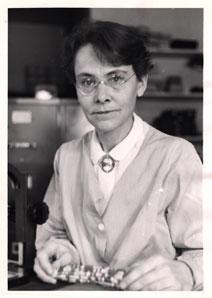
Figure 1: McClintock and her favorite research subject at Cold Spring Harbor.
- Mapping chromosomes
Johannsen named genes based on what they did, but he still didn't know what they were. The leading hypothesis when McClintock was growing up was that genes existed in some physical form on chromosomes , which were visible under the microscope as sausage-shaped blobs inside the nuclei of large cells , like the cells of maize. But even 20 years after genes were named, no one had yet demonstrated this.
While an instructor at Cornell University in the late 1920s, McClintock spent a lot of time describing chromosomes in maize cells . Maize (or corn) was the subject of a lot of scientific research at the time because it was an economically important plant, it was simple to cultivate, and it has easily observable traits ; these are some of the same reasons why Gregor Mendel studied pea plants nearly a century earlier (see our Scientists module for more on this topic).
McClintock made a map of each chromosome , describing features like a dark-looking knob at one end of chromosome 9 (Figure 2). McClintock and a graduate student named Harriet Creighton then compared the features in the chromosome maps with visible hereditary traits , like whether the kernels on the ears of corn were red or yellow, waxy or not. They saw a strong correlation between some chromosome locations and particular visible traits, and the same locations were always associated with the same traits in different corn kernels. Their interpretation of that correlation was that these locations on the chromosomes were the physical form of Mendel's "characters," the genes that had been named 20 years earlier by Johannsen. They convincingly demonstrated that when corn inherits a given feature in a specific location on a chromosome – a gene – it also inherits the trait associated with that gene (Creighton and McClintock, 1931).
Figure 2: Photomicrographs (a and b) of chromosomes from Zea mays , or maize. Diagrams of the chromosomes are shown in c and d. From (Coe and Kass, 2005).
McClintock and Creighton established that chromosomes are made up of multiple genes , lined up one after another, apparently in a stable order. Looking only at their results, it may not seem significant that they determined that the spot on maize chromosome 9 nearest the dark-looking knob determines kernel color; next to that, kernel shape; and farther from the knob, waxiness (Creighton and McClintock, 1931), but their work built on that of other scientists like Gregor Mendel and Charles Darwin (see our modules on Charles Darwin ) to develop a comprehensive theory of genetics and genetic inheritance .
Comprehension Checkpoint
- Changing mutation rates
McClintock had addressed the chromosome question by looking more closely at miniscule differences in maize better than anyone ever had. This attention to detail and her keen observational skills were essential to her work as a geneticist. She saw every irregularity in an ear of corn as a piece of evidence . Anything that didn't fit was something left to discover, and McClintock found that never-ending puzzle compelling. Having provided the best evidence yet for what genes are, hereditary units aligned along the chromosome like pearls on a necklace, she kept going, building on her work to make new discoveries.
In 1944, McClintock was elected to the National Academy of Sciences, one of the highest honors a scientist can receive. That same year, she noticed that a single leaf on one of the many maize plants she was growing at Cold Spring Harbor Laboratory in Long Island had a white streak (Figure 3). That was odd; the leaf was on a side branch and it ought to have been genetically identical to the main stalk, and thus solid green. McClintock wanted to get a closer look at what made that happen, so she performed an experiment by self-fertilizing the plant: collecting fresh powdery pollen from the tassel and shaking it onto the sticky, newly emerged silks on the ear. This commonly-used procedure promotes the appearance of recessive traits in the offspring.
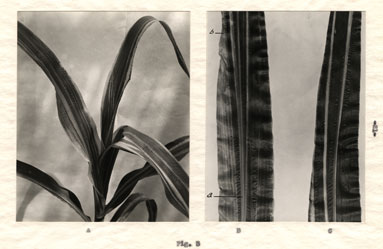
Figure 3: White streaking in maize leaf, from Barbara McClintock's papers.
The resulting ear of corn grew four different types of kernels. The most common was plain white and non-waxy, a type that she knew ought to produce plants with no mottling or streaking on their leaves. McClintock planted all forty of these white, non-waxy kernels on a seedling bench in her greenhouse in late winter to get an early start on the growing season. "Now the little seedlings that came up were extraordinary," she recalled, "About half of them seemed quite normal. And the other half were full of streaks" (Comfort, 2003). Some of the seedlings' leaves had wide green and wide yellow streaks, some were yellow with tiny little green streaks, some were green with spots of white.
McClintock knew that each spot or streak came from a "mutable gene": a gene that mutated as the plant was developing. But only two mutable genes had ever been described in maize before; among the offspring of the white-streak plant, McClintock was surprised to find seven or eight. She discussed her findings with a fellow geneticist then at Columbia University, Marcus Rhoades. With him, she shared her idea that these mutable genes must be more common than previously thought, but "they just have been neglected because no particular thought has been given to the appearance of changed sectors" (Fedoroff, 1998), or those spots and streaks. In fact, she thought that many plants that had exhibited these mutations might have been discarded from previous experiments as accidents that were a result of contamination (Comfort, 2003).
McClintock gave their appearance a lot of thought, and she had many discussions with Rhoades. She knew the details of her corn plants and their inheritance patterns better than anyone, and she knew that her experiments were not accidents. As a result of her extensive experience and background knowledge, McClintock was able to explain most of the mutable genes as variations of mechanisms she had already come up with in her earlier work. But one of them didn't make any sense. Usually, even though the gene mutated as cells divided and the plant grew, it mutated at a constant rate: A plant either had leaves with lots of streaks or leaves with just a few, but different leaves on the same plant shouldn't have different amounts of streaks or spots (Keller, 1983).
Plants bearing this odd mutable gene , though, did exhibit a varying mutation rate. A zone within a leaf could have more, or fewer, than the number of "expected" streaks. McClintock started to notice a pattern: One zone's mutation rate went up exactly as much as a neighbor's rate went down. Because of the way corn grows, McClintock knew that the pair of zones descended from a pair of cells , which began with the division of a single cell into two. "I couldn't get it out of my head that one cell gained what the other cell lost," she said (Keller, 1983).
- "Jumping" genes
What was it, then, that one cell gained? McClintock dropped all her other scientific projects and for the next six years grew descendants of that single side branch with the white streak on its leaf. She planned crosses, studied patterns of color on the leaves and kernels, and examined chromosomes under the microscope. She attended to every spot – sometimes to the point of seeing patterns were there were none, and laughing at herself in the morning. She also deeply respected the mysterious nature of creativity, and felt that sometimes the best thing she could do for her work was to go for a walk or sit under a tree. "When you suddenly see the problem, something happens that you have the answer – before you are able to put it into words. It is all done subconsciously" (Keller, 1983).
Her diligence, walks in the woods, and perseverance paid off. Eventually, McClintock showed that what one cell gained (and another lost) was a gene – a gene that had moved from one cell to another. This was a radical conclusion that flew in the face of the theory that she had helped to establish, a theory that described genes and chromosomes as stable entities. Genes were not supposed to move between cells. But this gene jumped. During a cell division, it removed itself from one chromosome and inserted itself into another. McClintock called these jumping genes transposons .
McClintock knew that her ideas would challenge the traditional notion of a stable genome . Genes that weren't just sitting inertly on a chromosome , but could move around, and didn't fit easily into the brand-new construct of a stable genome aligned along chromosomes. She thus waited until she had amassed enough evidence to present her work publicly, unveiling her intricate and complex hypothesis about transposons at the Cold Spring Harbor symposium of 1951 (McClintock, 1953). By that time, her results had been confirmed by other researchers at the University of Wisconsin, and though few in the audience understood her complex explanations, no one could refute it.
- The impact of transposons
Transposons turn out to be endlessly important. About half the human genome , in fact, is a graveyard of formerly jumping DNA (Mills et al., 2007). A little of it ( record , and scientists – everyone from molecular biologists to evolutionary geneticists to physicians – are still making progress in developing their understanding of the processes and consequences involved.
McClintock received the Nobel Prize in Physiology/Medicine in 1983 for her discovery of transposons. That discovery would not have been possible without her extremely detailed observations and well-planned experiments – she noticed even the smallest changes in individual kernels that she believed others had dismissed as contamination or error. She said that, "The important thing is to develop the capacity to see one kernel that is different, and make that understandable. If [something] doesn't fit, there's a reason, and you find out what it is" (Keller, 1983). In other words, a scientist should not automatically dismiss the unexpected or unusual, because that unusual thing might be the clue to a new discovery. McClintock enjoyed the puzzle that such unexpected observations presented, even though they challenged previously held notions (including her own). Over the course of her scientific career, McClintock's creativity and diligence allowed her to define – and redefine – the genes that had been postulated by other scientists before her.
Table of Contents
Activate glossary term highlighting to easily identify key terms within the module. Once highlighted, you can click on these terms to view their definitions.
Activate NGSS annotations to easily identify NGSS standards within the module. Once highlighted, you can click on them to view these standards.

IMAGES
COMMENTS
Creighlon and McClintock's experiment in corn to give proof for cytological crossing over. Using corn as the material, H.S. Creighton and Barbara McCIintock (1931) utilized the same principle which Stern utilized in case of fruitfly (Drosophila).
May 14, 2022 · Either a chromosome of normal length, but with a knob at one end, or an extra-long chromosome with no knob should be present. Creighton and McClintock found the latter, thus indicating that the gene locus for wx was associated with (and thus near) the end of the chromosome with the extra segment. The gene locus for kernel color must then be ...
The experiment of Creighton and McClintock, and the parallel experiment by Stern with Drosophila published in the same year, established the commonality in linear order between the chromosome and the genes within it. Only then could it be considered likely that the genetic material extended in a linear tract from one end of the chromosome to ...
Feb 9, 2017 · McClintock began her work on genetic recombination and transposition with doctoral student Harriet Baldwin Creighton in 1931 at Cornell's College of Agriculture in Ithaca, New York. Together they examined maize chromosomes and published their results in the 1931 paper "A Correlation of Cytological and Genetical Crossing-Over in Zea Mays ."
Barbara McClintock was a pioneer in the field of cytogenetics, and she left a lasting legacy of superb experimental inquiry. McClintock’s breeding experiments with maize are particularly notable ...
May 10, 2005 · Harriet Creighton (1909-2004) and Barbara McClintock (1902-1992) [Creighton, H. B. & McClintock, B. (1931) Proc. Natl. Acad. Sci. USA 17, 492-497] showed by an elegantly simple experiment in 1931 that exchange between genes was accompanied by exchange of cytological, i.e., physical, parts of chromosomes.
But this still is not definitive proof. That crossing over was the result of an exchange of genetic information between homologous chromosomes was definitively shown by the experiments initially performed by Harriet Creighton and Barbara McClintock (1931). They used a combination of genetic and cytological evidence in their proof.
Harriet Creighton (1909-2004) and Barbara McClintock (1902-1992) [Creighton, H. B. & McClintock, B. (1931) Proc. Natl. Acad. Sci. USA 17, 492-4971 showed by an elegantly simple experiment in 1931 that exchange between genes was accompanied by exchange of cytological, i.e., physical, parts of chromosomes.
McClintock and Creighton established that chromosomes are made up of multiple genes, lined up one after another, apparently in a stable order.Looking only at their results, it may not seem significant that they determined that the spot on maize chromosome 9 nearest the dark-looking knob determines kernel color; next to that, kernel shape; and farther from the knob, waxiness (Creighton and ...
bacteria two decades later that McClintock's work was truly appreciated. McClintock won the Nobel Prize for her work on transposable elements in 1983. The maize life cycle and kernel development A single corn plant contains both male and female germlines and is capable of self or cross fertilization. Fertilization begins when a pollen grain ...